Admin مدير المنتدى


عدد المساهمات : 18992 التقييم : 35482 تاريخ التسجيل : 01/07/2009 الدولة : مصر العمل : مدير منتدى هندسة الإنتاج والتصميم الميكانيكى
 | موضوع: كتاب Biologically Inspired Control of Humanoid Robot Arms الثلاثاء 18 مايو 2021, 2:34 am | |
| 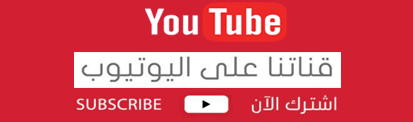
أخوانى فى الله أحضرت لكم كتاب Biologically Inspired Control of Humanoid Robot Arms Robust and Adaptive Approaches Adam Spiers • Said Ghani Khan • Guido Herrmann
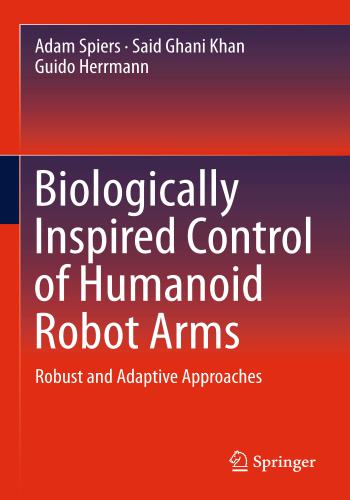 و المحتوى كما يلي :
Contents 1 Introduction . 1 1.1 Prologue 1 1.1.1 Industrial Robots . 2 1.1.2 Humanoid Robots 4 1.1.3 The Importance of Human-Like Motion 5 1.1.4 Biologically Inspired Design . 6 1.1.5 Physical Safety and Active Compliance for Safety . 6 1.1.6 Robust and Adaptive Control 7 1.2 Objective of the Book 7 1.3 Guidance for the Reader . 8 1.3.1 Recommended Reading Routes 9 References 10 Part I Background on Humanoid Robots and Human Motion 2 Humanoid Robots and Control . 15 2.1 Humanoid Robots 15 2.1.1 Functional Tools 16 2.1.2 Models of Humans . 17 2.1.3 Human–Robot Interaction 17 2.2 Goals of Human-Like Motion . 18 2.3 Robot Motion Control Overview 19 2.3.1 Kinematics-Based Robot Motion Control . 21 2.3.2 Dynamic-Based Robot Motion Control . 25 2.3.3 Optimal Control 28 2.3.4 Operational Space Control . 29 2.3.5 Dual Robot Arm Control . 30 2.3.6 Hand Grasping Control . 31 2.4 Sensing and Robot Arm Motion . 32 xixii Contents 2.5 Robot and Control Hardware 33 2.5.1 Elumotion Robotic Platform . 34 2.5.2 Robot Structure . 37 2.5.3 Actuators 39 2.5.4 Motor Drivers . 39 2.5.5 EPOS Interface method 40 2.6 Summary 41 References 41 3 Human Motion 49 3.1 Introduction . 49 3.2 Motion Studies . 50 3.3 Motion Models . 51 3.3.1 Kinematic Models 51 3.3.2 Dynamic Models 53 3.4 Physiological Modelling . 55 3.4.1 Muscle Models 55 3.4.2 Physiological Complexity 56 3.4.3 Neural Models 57 3.4.4 Simplified Models 59 3.5 Motion Capture Methods and Technology 59 3.6 Human Motion Reproduction and Synthesis 63 3.6.1 Direct Reproduction of Human Motion . 63 3.6.2 Learning Techniques . 66 3.6.3 Dynamic Movement Primitives 67 3.6.4 Operational Space Control . 68 3.7 Summary 68 References 70 Part II Robot Control: Implementation 4 Basic Operational Space Controller 77 4.1 Introduction . 77 4.1.1 Human Verification . 78 4.1.2 Robot Specification . 79 4.1.3 Robot Goal Modification . 81 4.2 The Operational Space Mathematical Formulation . 81 4.3 Task Control 82 4.3.1 Jacobian Pseudo Inverse 82 4.3.2 Task-Space Dynamic Projection . 83 4.3.3 Feedback Linearisation . 85 4.4 Posture Control . 86 4.4.1 ‘Effort’ Cost Function 86 4.4.2 Task/Posture Isolation 89Contents xiii 4.5 Simulation and Implementation . 90 4.5.1 Controller Realisation 90 4.5.2 Simulation Results 92 4.5.3 Robot Implementation 97 4.6 Summary 99 References 100 5 Sliding Mode Task Controller Modification . 101 5.1 Introduction . 101 5.2 Sliding Mode Control Overview . 102 5.3 Controller Design 103 5.3.1 Switching Function . 103 5.3.2 Variable Structure Law . 105 5.4 Lyapunov Stability Analysis . 107 5.5 Results 108 5.5.1 Simulation 108 5.5.2 Physical Robot 111 5.5.3 PID Results . 111 5.5.4 Sliding Mode Results . 112 5.5.5 Demand Filter . 113 5.6 Compliance . 114 5.7 Summary 114 References 115 6 Implementing ‘Discomfort’ for Smooth Joint Limits . 117 6.1 Introduction . 117 6.1.1 Dynamic Model Simplicity 118 6.2 Visualisation Technique 119 6.2.1 Motion Analysis 121 6.3 Joint Limit Function Design . 122 6.3.1 Integration with the Effort Function . 123 6.4 Results 124 6.4.1 Simulated Results . 124 6.4.2 Practical Results 126 6.5 Summary 128 References 130 7 Sliding Mode Optimal Controller . 131 7.1 Introduction . 131 7.2 Controller Design 132 7.2.1 Optimal Sliding Surface 132 7.2.2 Control Method . 135 7.2.3 Velocity Decoupling 138 7.2.4 Overall Controller 144 7.3 Implementation Issues: Viscous Friction Identification and Compensation 146xiv Contents 7.4 Simulated Implementation . 147 7.4.1 Controller Effort 149 7.4.2 Friction Model 151 7.4.3 Simulated Results . 151 7.5 Practical Implementation 156 7.6 Summary 158 References 159 8 Adaptive Compliance Control with Anti-windup Compensation and Posture Control 161 8.1 Introduction . 161 8.2 Adaptive Compliance Control for Task Motion 163 8.2.1 Impedance Reference Model . 165 8.2.2 Principle of the Model Reference Scheme 166 8.3 Effort-Minimising Posture Torque Controller 167 8.4 Anti-windup Compensator . 168 8.5 Implementation . 171 8.6 One-Dimensional Adaptive Compliance Control of a Robot Arm . 171 8.6.1 Tracking . 171 8.6.2 Compliance Results 173 8.6.3 Anti-windup Compensator Results 174 8.7 Multidimensional Adaptive Compliance Control of a Robot Arm. 181 8.7.1 Joint Torque Sensors and Body Torque Estimates 182 8.7.2 Tracking and Compliance Results . 185 8.7.3 Anti-windup Compensator Results 185 8.8 Summary 190 References 190 Part III Human Motion Recording for Task Motion Modelling and Robot Arm Control 9 Human Motion Recording and Analysis . 195 9.1 Initial Motion Capture Objective 195 9.1.1 The Vicon System 199 9.1.2 Experimental Set-up 200 9.1.3 Results 201 9.1.4 Summary of Initial Motion Capture Experiments . 203 9.2 Motion Capture for Robotic Implementation . 206 9.2.1 Human–Robot Kinematic Mismatch 206 9.2.2 Motion Capture Process for Inconsistent Kinematic Models 208 9.2.3 Extended Motion Capture Method . 210 9.2.4 Vicon Skeleton Model 212 9.2.5 Incompatible Kinematics Removal 213Contents xv 9.2.6 Inverse Kinematics . 215 9.2.7 Trajectory Discrepancy . 217 9.3 Four Degrees of Freedom Comparative Trials 218 9.3.1 Results 219 9.4 Summary 221 References 222 10 Neural Network Motion Learning by Observation for Task Modelling and Control . 225 10.1 Introduction . 225 10.1.1 Learning by Observation . 226 10.2 Learning by Observation Method . 228 10.3 Minimal Trajectory Encoding . 229 10.3.1 Polynomial Encoding Issues . 229 10.3.2 Scaling and Fitting of Generated Trajectories . 231 10.4 Network Structure 233 10.5 Experimental Procedure 234 10.5.1 Sub-motion Splitting . 235 10.5.2 Training Data . 235 10.5.3 Neural Network Results 237 10.6 Integration into the Robot Controller 241 10.7 Summary 245 References 245 Appendix A Kinematics: Introduction . 247 A.1 Kinematics Notation . 247 A.1.1 Position Vector 248 A.1.2 Rotation Matrix . 249 A.1.3 Transformation Matrix . 251 A.2 Denavit–Hartenberg Notation . 251 A.2.1 Frame Assignment Convention 252 A.2.2 DH Parameters 252 A.3 Applied Kinematics 253 A.3.1 Forward Kinematics 253 A.3.2 Inverse Kinematics . 254 A.4 Robot Jacobian . 254 References 255 Appendix B Inverse Kinematics for BERUL2 257 B.1 Denavit–Hartenberg Parameters . 257 B.2 Forward Kinematics 257 B.3 Algebraic Solution . 258 Reference . 264xvi Contents Appendix C Theoretical Summary of Adaptive Compliant Controller 265 C.1 Proof of Theorem 1 265 References 269 Appendix D List of Videos 271 Index . Index A Activation Matrix, 87, 111 Actuator Dynamics, 98 Actuator Saturation, 138, 163, 168 Adaptation, 226 Adaptive, 161 Adaptive Compliance Control, 163 Adaptive Control, 7, 21, 68, 163 Anatomical Landmarks, 213 Antagonism, 56, 87 Anthropomorphic, 18 Anti-Windup Compensator, 161, 163, 168, 174, 185 Approach and Adjustment, 51 AW-compensator, 265 B Balance, 54 Bang-Bang Control, 106 Bell-shaped velocity profiles, 51, 52 BERT, 34, 97 BERT/BERUL Actuators, 39 BERT2, 36, 162 BERUL, 34, 90, 97, 111, 126, 146, 156 BERUL2, 36, 126, 195, 206, 241, 257 Biologically Inspired, 5, 6, 19, 130 Biomechanical, 5 Boundary Layer, 145, 152 Branched Systems, 27 C CAN, 39 Cartesian Workspace, 23 Centrifugal, 26, 81, 84 Chattering, 106 Co-ordinate Frame, 248 Co-ordinate System, 20 Compliance, 6, 24, 67, 114, 161, 162, 173 Compliance Control, 162, 163 Confidence, 5 Controller Effort, 149 Convergence, 161 Coordinate Frame, 248 Coriolis, 26, 81, 84 Cost Function, 78, 123, 141 Cost Gradient, 122 Coulomb Friction, 151 Curved Motion Trajectories, 52 D Damaging Hardware, 117 Danger, 131 Decoupling, 139 Decoupling Term, 89 Demand Filter, 113 Denavit–Hartenberg, 251, 257 Discomfort, 79, 122, 123, 130 Disturbance, 114, 131 dSPACE, 39, 90 Dual Arm Control, 30 Dynamic Control, 2 Dynamic Model, 7, 118 Dynamic Modelling, 26 Dynamic Models of Human Motion, 53 Dynamic Motion Primitives, 67 Dynamic Muscle Modelling, 59 Dynamics, 164
Springer International Publishing Switzerland 2016 A. Spiers et al., Biologically Inspired Control of Humanoid Robot Arms, DOI 10.1007/978-3-319-30160-0 273274 Index E Effort, 86, 87, 225 effort, 124 Effort Function, 86, 93, 119, 123 Effort Minimisation, 96, 97, 132, 147 Effort Optimisation, 227 Effort Plot, 120 Effort Profiles, 93 Effort Visualisation, 119 Elbow Configuration, 119 Elumotion, 34, 111 Encoders, 111 EPOS, 40, 90 Equilibrium Point Hypothesis, 52 Evolution, 6 Exoskeleton, 58 F Feedback Linearisation, 77, 85, 92, 104, 134, 147 Filter, 113 Fitt’s Law, 51 Fitting, 231 Force Control, 6, 20, 81, 85 Forgetting Factor, 165 Forward Kinematics, 22, 253, 257 Friction, 146 Friction Compensation, 134, 146 Friction Model, 151 G Goals of Human-Like Motion, 18 Gravity, 26, 52, 53, 197, 200, 227 Gravity Compensation, 146 H Harmonic Drive, 98, 146 Hazardous Environments, 4 Hierarchical Control, 68 Hill Equation, 55 Human Arm, 16 Human Behaviour, 17, 18 Human Motion, 49, 197 Human Motion Models, 50, 51 Human Motion Synthesis, 15 Human–Human Interaction, 20 Human–Robot Interaction, 4, 6, 17, 24, 68, 131, 138, 159, 161, 226 Human-Like Appearance, 5 Human-Like Motion, 5, 18 I Imitation, 207 Impedance Control, 162 Implementation, 90 Inconsistent Kinematic Models, 208 Industrial Robotics, 2, 6, 18, 24 Input Passive, 269 Instability, 20, 68 Inverse Kinematics, 2, 20, 22, 119, 215, 254, 257 J Jacobian, 82, 111, 139, 254 Jacobian Pseudo-Inverse, 77, 82, 139, 164 Jerk, 52 Joint Level Control, 20 Joint Limits, 65, 117, 122, 132, 152 Joint Space, 23 Joint Space Dynamics, 83 K Kinematic Mismatch, 206 Kinematic Models of Human Motion, 51 Kinematics, 247 Kinematics Notation, 247 KUKA, 21 L Lagrangian, 26 Lagrangian Dynamics, 85 Learning, 19, 66, 225, 226 Learning by Demonstration, 226 Learning by Observation, 225, 226, 228 Levenberg-Marquardt algorithm, 233 Limit Switch, 117, 126 Local Stability, 138 Lyapunov Analysis of Sliding Surface, 134 Lyapunov Function, 131, 135, 266 Lyapunov Methods, 132 Lyapunov Stability, 107, 161 Lyapunov Theory, 132 M Maple, 85, 90 MATLAB, 90, 234 Metabolic, 55, 56, 87 Minimal Energy Criteria, 54 Model Mismatch, 7, 111, 150, 152 Model Reference Scheme Principle, 166 Motion Capture, 59, 195, 199, 200, 206, 227, 234Index 275 Motion Priority, 82 Motion Studies, 50 Motor Driver, 39 MRAC - Model Reference Adaptive Control, 162, 174 Muscle, 18, 53, 87 Muscle Control, 51 Muscle Dynamics, 56 Muscle Effort, 79 Muscle Matrix, 87, 88, 92, 125 Muscle Modelling, 57 Musculoskeletal System, 88 Muybridge, 50 N Neural Models of Motion, 57 Neural Network, 163, 225, 233 Non-linear System, 20 Non-Monotonic, 52, 78 Normalisation, 229 Null Space, 81, 89, 165, 269 O Objectives of Humanoid Robots, 1 Operational Space, 68, 77, 81, 89, 104, 138, 143 Operational Space Control, 29, 131 Optimal Control, 28 Optimal Controller, 86, 128 Optimality in Robot Motion, 25 Optimisation, 52, 53, 77, 132 Optimisation in Human Motion, 2 Overhead Reaching Task, 78 P Pain, 123 Passivity, 161, 269 PD, 109, 138 Pectoral Girdle, 214 Perceptron, 233 Phase Plane, 109 Physical Safety, 6 Physiological, 198, 225, 227 Physiology, 55, 207, 227 PID Control, 111 Planar Robot, 23, 78 Polynomial Encoding, 228, 229 Position Vector, 248 Posture, 21, 77, 87 Posture Controller, 82, 86, 117 Posture Space Dynamics, 268 Posture Torque Controller, 167 Potential Field, 117, 122, 128 Projection, 84 R Reaching Motion, 49, 77, 78, 110, 125, 197, 200, 203, 227 Reaching Phase, 102 Recursive Newton-Euler Algorithm, 26 Redundancy, 20, 22 Redundancy Problem, 50, 54 Reference Model, 165 Reinforcement Learning, 67 Robot Dynamics, 20 Robot Motion Control, 19 Robot Structure, 37 Robust, 105 Robust Control, 7, 21, 68 Robustness, 131, 165, 269 Rotation Matrix, 249 S S-function, 90 Safety, 2, 4, 6, 24 Safety Critical, 131 Saturation, 269 Saturation Function, 151 Scaling, 231 Scheduling Element, 168, 189 Shoulder, 207 SimMechanics, 87, 90 Simulations, 18 Simulink, 90, 151 Singularity, 111, 121 Sliding Mode Control, 7, 102, 108, 109, 131 Sliding Optimal, 147 Sliding Optimal Controller, 131, 132, 144, 147, 220 Sliding Phase, 102 Sliding Surface, 102, 106, 109, 132, 133 Social Robotics, 17 Social Robots, 161 Spatial Notation, 27 Spline Curve, 23 Stability, 105, 165, 269 Stability Condition, 145 Stability Proof, 265 Static Friction, 147 Steepest Descent, 132, 133, 142 Stiffness Matrix, 165 Straight Line Motion Trajectories, 52 Switching Control, 132276 Index Switching Function, 103 Synthetic Motion, 77 System Identification, 146 T Task Control, 85 Task Controller, 82, 85, 103 Task Space, 81 Task Space Projection, 84 Task/Posture Decoupling, 138 Tendon, 56 Tool Use, 16 Torque Metric, 149, 153 Torque Sensors, 36 Tracking, 171, 241 Training, 235 Transformation Matrix, 251 U Ultimate Bounded Stability, 165 Ultimately Bounded Stable, 265, 269 Un-modelled Dynamics, 28 Uncertainty, 102, 105, 131, 136, 137, 151 Unmodelled Dynamics, 98 Unnatural Posture, 117 V Variable Structure, 105 Vertical Reaching, 52 Vicon, 64, 199, 212, 227, 234, 259 Viscous Friction, 134, 146 Visualisation, 119 VITE model, 51 W Windup, 163 Work Cell, 2, 3, 18 Z Zero-Gravity, 146
كلمة سر فك الضغط : books-world.net The Unzip Password : books-world.net أتمنى أن تستفيدوا من محتوى الموضوع وأن ينال إعجابكم رابط من موقع عالم الكتب لتنزيل كتاب Biologically Inspired Control of Humanoid Robot Arms رابط مباشر لتنزيل كتاب Biologically Inspired Control of Humanoid Robot Arms 
|
|